- Home >
- Learning centre >
- Science blog >
- Bacteria in Biofilms: United They Stand, Divided They Fall
Bacteria in Biofilms: United They Stand, Divided They Fall
10th August 2023
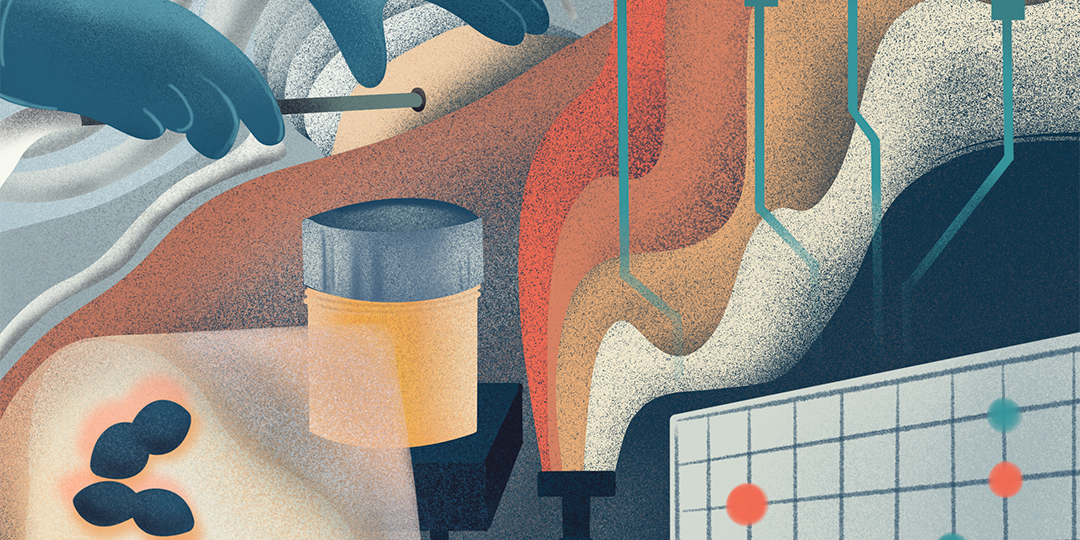
While best known for its applicability to human unity, this phrase also holds true to microbes.
Like humans, replicating microbes that adhere to a surface built themselves homes of a gelatinous, insoluble material. These three-dimensional communities are called biofilms.
Biofilms can form on wet surfaces in different settings by any type of microbe, such as bacteria, fungi, and algae. Examples of biofilms range from slime on pond rocks to dental plaque. They can form in rainforests, hot deserts, and Antarctica. They exist on both plant and animal tissues.
A community of microbes growing on an outdoor object may hardly seem like a concern. However, when the surface colonized is a medical device or human body, all sorts of problems can arise.
This is precisely what happens in hospitals across the world. Hospital-acquired infections claim 2 million lives annually in the United States alone (Wenzel, 2007). Of these, 60-70% can be attributed to biofilms on implanted medical devices (NIH Source).
Beyond devices, biofilms can also form in the lungs of cystic fibrosis patients, on the bones of osteomyelitis patients, in the ears of otitis media patients, and in the mouths of periodontitis patients. Bacteria belonging to the Escherichia, Listeria, Mycobacteria, Pseudomonas, Streptococcus, Salmonella, and Vibrio species are the commonly identified in infectious biofilms, but fungal species such as Candida albicans also pose a serious threat.
The fact that bacteria can wreak havoc on a person’s body is well-known. However, biofilms pose a unique threat. First, the thick matrix protects its inhabitants from immune cells and antimicrobial drugs such as antibiotics. Second, the microbes within the community communicate with each other through a process known as quorum sensing. This can lead to the collective increase of virulence factors. Finally, some cases end in thromboembolism when part of the biofilm detaches and clogs a blood vessel. This condition is life-threatening.
In short, biofilms can kill.
Unfortunately, most of the research done on microbes has been done on individual cells and not in the collective biofilm setting. This has led to a poor understanding of what to expect with clinical samples including an overestimation on the efficacy of antibiotics. Furthermore, the assessment of drug susceptibility is done by measuring the amount of bacteria growing in suspension or forming individual colonies on an agar plate–methods that do not account for the complexities of community interactions as seen in biofilms. These assays have low reproducibility and can be labor-intensive.
The only known treatments for medical biofilms are antibiotics and removal of contaminated medical devices. However, this does not guarantee clearance of medical device-induced infection and is not for biofilms which have formed independently of medical devices (such is the case with cystic fibrosis patients).
Biofilms help microbes escape antibiotics in several ways. For instance, the gelatinous material that makes up the extracellular matrix traps and/or neutralizes some antimicrobials, increasing the minimum inhibitory concentration (MIC) needed for the drug to take effect. This means that a concentration of an antibiotic shown to be effective on single bacteria in the lab will not kill the same bacteria in the biofilm since the amount of active drug reaching those bacteria will not be enough to kill them.
In other cases, even if the concentration of the antibiotic is high enough to be toxic to the bacteria it reaches, the existence of microbes within a community means that some members can be metabolically dormant. Since antibiotics target active pathways in bacterial replication, they generally do not kill inactive bacteria. These “persisters” are thus resistant to many antibiotics.
Current research strategies to combat biofilm resistance include compounds that can reactivate dormant bacteria and blocking the communication between bacteria which help them build their communities. Reactivating dormant bacteria makes them once again susceptible to antibiotics. Blocking quorum sensing can lead to disruption of the biofilm and dispersal of more susceptible and less virulent planktonic forms of the bacteria.
One method being used to measure the susceptibility of medical biofilms to drugs is called the calorimetry-based biofilm susceptibility (CBS) assay. This assay uses a microcalorimeter to track the heat output of intact biofilms in real-time. Since active microbes have higher metabolisms than inactive ones, CBS assays can track reduction of metabolism in active microbes or the reactivation of dormant cells by drugs.
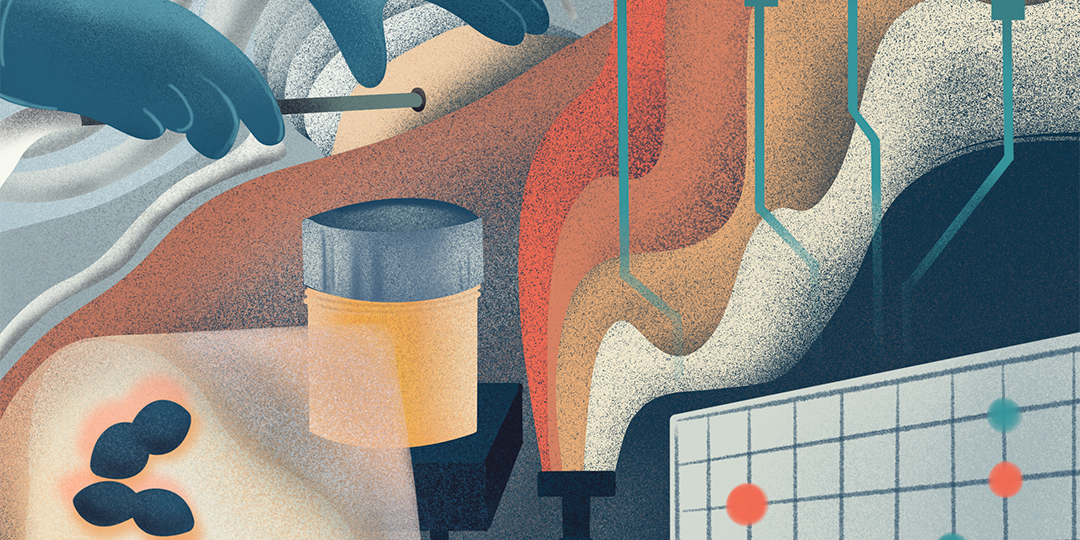
STAY UP-TO-DATE
Subscribe to our newsletter and stay up to date with the latest news and updates